Advancing Translational Research with RMP1-14: From PD-1 Pathway Insights to Clinical Applications
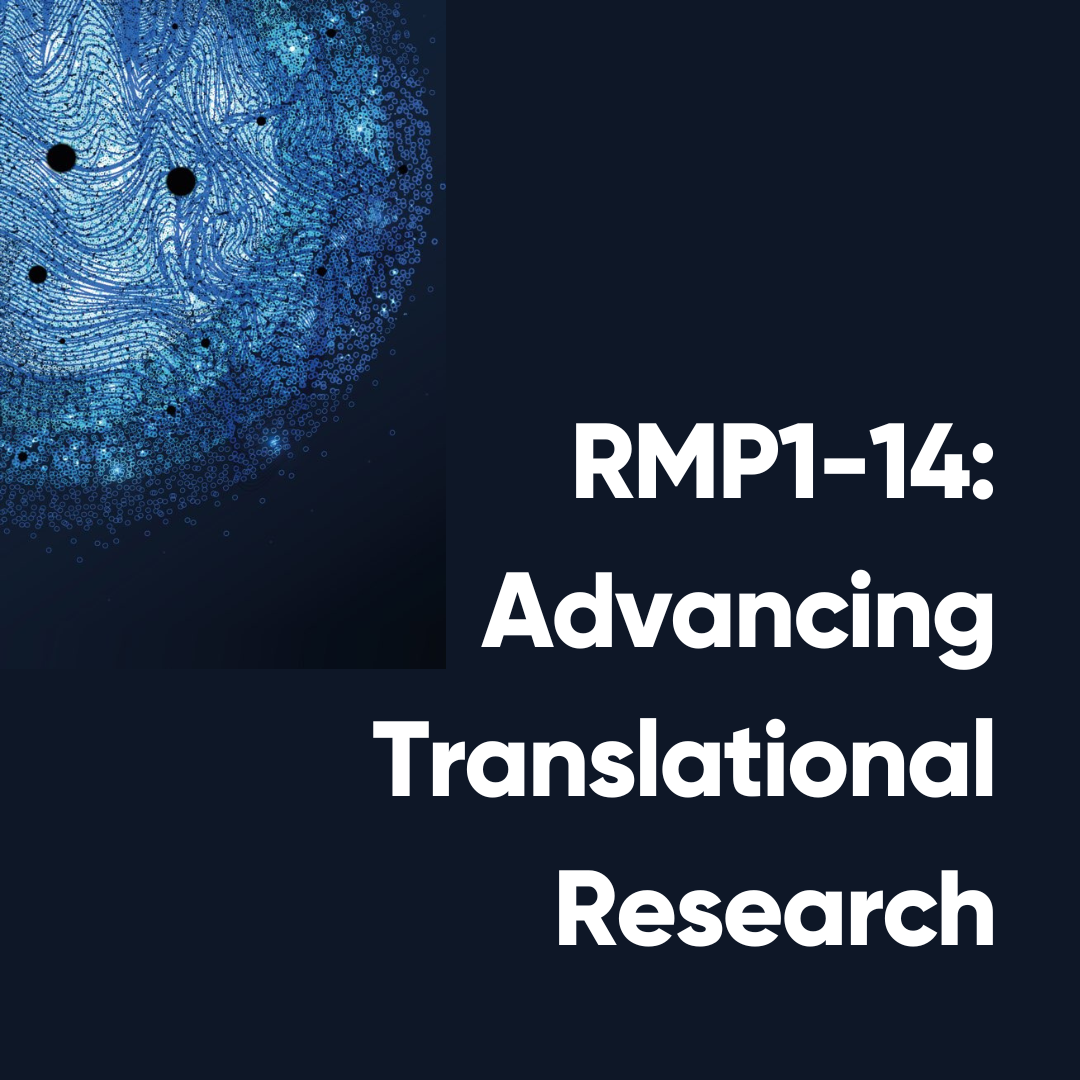
Introduction
In advancing cancer immunotherapy, antibodies against PD-1 have become a breakthrough and opened a new door for severely affected cancer patients. Nevertheless, the results of preclinical studies have faced various obstacles in transforming into successful clinical applications, requiring a solid translational research channel with profound learning of fundamental biology [1]. In this review, we will discover the significant role of RMP1-14 as an anti-PD-1 antibody for the expanding understanding of the PD-1 pathway and improving clinical policies, emphasizing its functions as a link between taking care of patients and simple science.
Revealing PD-1 Pathway Biology with RMP1-14
The heart of translational research is an essential learning of the biological processes and principles of action that direct diagnosis and treatment-based outcomes. Regarding the PD-1 pathway, the RMP1-14 antibody has clarified related immune checkpoints that regulate T-cells’ functions, differentiation, and activations [2].
According to preclinical investigations of RMP1-14, Researchers have studied the insightful association between PD-1 and related ligands (PD-L1 and PD-L2), including T-cell regulation via downstream signaling. For instance, PD-1 and its Ligands complex activate SHP-2 (the SH2 domain-containing protein tyrosine phosphatase-2), which lessens T cell proliferation and activation with its signaling receptor [3, 4].
The antibody RMP1-14 has been investigated to reveal the effects of PD-1 on memory formation and T cell differentiation by explaining PD-1’s functions in CD8+ cytotoxic T cells and CD4+ helper T cells. Recent studies reported that the policies designed for treatment modulated the PD-1 signal, which was learned entirely of regulation in T cell biology [5, 6]. Moreover, the RMP1-14 antibody has been studied to explore the association between PD-1, CTLA-4, and LAG (other immune checkpoints) to explore possible synergistic and antagonistic impacts of immunotherapies, selecting optimal targets and periods of interference [7].
Preclinical Models and Translational Applications
Preclinical models play a critical role in translating fundamental biological insights into potential clinical applications, and RMP1-14 has been extensively used in various animal models to demonstrate the therapeutic potential of PD-1 blockade [8].
RMP1-14 has stimulated anti-tumor activity, prevented tumor growth, and enhanced survival rate, along with the impact of PD-1 inhibition in treating cancer-immune diseases in mouse models. These studies have also helped identify the tumor types and stages most likely to respond to PD-1 blockade, informing the selection of clinical indications for further development [9].
The efficiency of PD-1 inhibitors in conjugation with other medication methods, including radiation therapy, targeted therapies, and chemotherapy, depends on RMP1-14 studies. So, the strategies of clinical studies are to assess combined treatments and inform possible therapies between PD-1 blockade and traditional medications through these investigations [10, 11].
Furthermore, preclinical research employing RMP1-14 antibodies has developed several animal models for clinical trials, such as patient-derived xenografts and humanized mouse models, representing the simple form of tumor biology and related immune responses. These models have enabled the evaluation of PD-1 blockade in the context of human immune cells and have facilitated the translation of preclinical findings to clinical settings [12].
Additionally, the RMP1-14 antibody has been utilized to investigate the effects of PD-1 inhibitors in several diseases, including autoimmunity and infectious diseases. According to recent research, MRP1-14 also stimulates anti-viral activity, leading to pathogen clearance and possible implementations in HIV and hepatitis. Similarly, RMP1-14 has been used to investigate the role of PD-1 in maintaining immune tolerance and preventing autoimmunity, providing insights into the potential risks and benefits of PD-1 inhibition in these settings [13, 14].
Supporting Clinical Development and Decision-Making
Beyond its preclinical applications, RMP1-14 has been crucial in supporting clinical development and decision-making for PD-1 targeted therapies. The wealth of preclinical data generated using RMP1-14 has provided a robust foundation for designing and interpreting clinical trials, helping bridge the gap between bench and bedside.
RMP1-14-based preclinical investigations have provided guidelines for standard doses, ways of administration, and time management for clinical aspects. These recommendations induce the development of proper protocols and explain clinical data by illustrating PD-1 inhibitors of pharmacodynamics, pharmacokinetics, and safety issues.
The studies of the RMP1-14 antibody have offered possible biomarkers and predictors of responses associated with PD-1 inhibitors, including immune cell filtration, tumor mutational burden, and PD-L1 expression. These results provide accurate diagnostic and patient stratification procedures for clinical studies, separating privileged populations due to PD-1 targeted therapies.
In addition, RMP1-14 antibody studies have presented relative statistics, which allow for the assessment of PD-1 blockade’s efficiency and security and display for the assortment of principal aspirants for clinical research. By benchmarking the performance of RMP1-14 against other anti-PD-1 clones and clinical-stage antibodies, these studies have delivered crucial context for understanding clinical trial results and have educated strategic decision-making for drug development programs.
Case Studies and Success Stories
To illustrate the impact of RMP1-14 in advancing translational research and informing clinical development, let's highlight a few notable case studies and success stories from the literature.
Nivolumab is one of the earlier approved drugs for PD-1 blockade, while the RMP1-14 antibody’s main contribution is understanding the impacts of PD-1 inhibitors and designing a primary basement for Nivolumab-based clinical assessment in several tumor models. The success of Nivolumab in clinical trials, leading to its approval for multiple indications, including melanoma, non-small cell lung cancer, and renal cell carcinoma, can be traced back to the pioneering work with RMP1-14 in preclinical settings [15, 16].
Another extensively industrialized drug as a PD-1 blockade is Pembrolizumab. RMP1-14-based studies offered the proper mechanism of PD-1 inhibitors, including assessing relative statistics of Pembrolizumab’s efficiency and security. The insights from these studies informed the design of clinical trials and supported Pembrolizumab’s successful development and approval for various indications [17].
In addition to supporting the development of individual drugs, RMP1-14 has also been instrumental in advancing our understanding of the biology and therapeutic potential of the PD-1 pathway. For example, preclinical studies using RMP1-14 have shed light on the complex interactions between PD-1 and other immune checkpoints, such as CTLA-4, and have guided the development of combination immunotherapy strategies. The success of combination regimens, such as nivolumab plus ipilimumab, in clinical trials can partly be attributed to the foundational work with RMP1-14 in elucidating the underlying mechanisms and potential synergies [18, 19].
Conclusion
RMP1-14 has been vital in advancing translational research and bridging the gap between basic science and clinical applications in immuno-oncology. By providing a powerful means to investigate the biology of the PD-1 pathway, evaluate therapeutic strategies in preclinical models, and inform clinical development and decision-making, RMP1-14 has played a central role in the success of PD-1 targeted therapies.
As we continue to unravel the complexities of the immune system and develop new approaches to harness its power for fighting disease, tools like RMP1-14 will remain indispensable. By leveraging the insights gained from preclinical studies and translating them into clinical innovations, we can accelerate the pace of discovery and bring new hope to patients in need.
Looking forward, there are still many exciting opportunities and challenges ahead for RMP1-14 and the broader field of immuno-oncology. We will uncover new insights and therapeutic possibilities as we deepen our understanding of the PD-1 pathway and its role in various diseases. Researchers will continue to rely on RMP1-14 as a valuable tool for interrogating the biology of PD-1 and evaluating novel strategies for modulating its activity.
At the same time, the success of RMP1-14 and other preclinical tools will depend on our ability to translate their findings into clinical practice effectively. This will require ongoing collaboration between basic scientists, clinical researchers, and industry partners to ensure that the most promising strategies are efficiently developed and tested in human trials.
Ultimately, the story of RMP1-14 is one of scientific curiosity, perseverance, and the power of translational research to transform medicine. By building on the foundation laid by this remarkable antibody and the dedicated researchers who have studied it, we can continue to push the boundaries of what is possible and bring new hope to patients worldwide.
Are you seeking reasonable, high-quality RMP1-14 antibodies to further your research? Ichorbio has an extensive reputation for providing various stages of high-grade RMP1-14 antibodies, such as low endotoxin, ultra-low endotoxin, extremely low endotoxin, murinized versions of RMP1-14, and Fc silenced RMP1-14.
To find our RMP1-14 Products: https://ichor.bio/rmp1-14
References:
1. Gong J, Chehrazi-Raffle A, Reddi S, Salgia R. Development of PD-1 and PD-L1 inhibitors as a form of cancer immunotherapy: a comprehensive review of registration trials and future considerations. J Immunother Cancer. 2018 Jan 23;6(1):8.
2. Boussiotis VA. Molecular and Biochemical Aspects of the PD-1 Checkpoint Pathway. N Engl J Med. 2016 Nov 3;375(18):1767-1778.
3. Galván Morales MA, Montero-Vargas JM, Vizuet-de-Rueda JC, Teran LM. New Insights into the Role of PD-1 and Its Ligands in Allergic Disease. Int J Mol Sci. 2021 Nov 2;22(21):11898.
4. Ghosh C, Luong G, Sun Y. A snapshot of the PD-1/PD-L1 pathway. J Cancer. 2021 Mar 5;12(9):2735-2746.
5. Konkel JE, Frommer F, Leech MD, Yagita H, Waisman A, Anderton SM. PD-1 signalling in CD4(+) T cells restrains their clonal expansion to an immunogenic stimulus, but is not critically required for peptide-induced tolerance. Immunology. 2010 May;130(1):92-102.
6. Pauken KE, Godec J, Odorizzi PM, Brown KE, Yates KB, et al. The PD-1 Pathway Regulates Development and Function of Memory CD8+ T Cells following Respiratory Viral Infection. Cell Rep. 2020 Jun 30;31(13):107827.
7. Huang RY, Francois A, McGray AR, Miliotto A, Odunsi K. Compensatory upregulation of PD-1, LAG-3, and CTLA-4 limits the efficacy of single-agent checkpoint blockade in metastatic ovarian cancer. Oncoimmunology. 2016 Oct 28;6(1): e1249561.
8. Zheng Y, Zhang H, Xiao C, Deng Z, Fan T, Zheng B, Li C, He J. KLF12 overcomes anti-PD-1 resistance by reducing galectin-1 in cancer cells. J Immunother Cancer. 2023 Aug;11(8): e007286.
9. Jin Y, An X, Mao B, Sun R, Kumari R, Chen X, et al. Different syngeneic tumors show distinctive intrinsic tumor-immunity and mechanisms of actions (MOA) of anti-PD-1 treatment. Sci Rep. 2022 Feb 28;12(1):3278.
10. Hong S, Bi M, Yu H, Yan Z, Wang H. Radiation therapy enhanced therapeutic efficacy of anti-PD1 against gastric cancer. J Radiat Res. 2020 Nov 16;61(6):851-859.
11. Liu L, Huang X, Shi F, Song J, Guo C, Yang J, Liang T, Bai X. Combination therapy for pancreatic cancer: anti-PD-(L)1-based strategy. J Exp Clin Cancer Res. 2022 Feb 9;41(1):56.
12. Jin KT, Du WL, Lan HR, Liu YY, Mao CS, Du JL, Mou XZ. Development of humanized mouse with patient-derived xenografts for cancer immunotherapy studies: A comprehensive review. Cancer Sci. 2021 Jul;112(7):2592-2606.
13. Chen RY, Zhu Y, Shen YY, Xu QY, Tang HY, Cui NX, Jiang L, Dai XM, Chen WQ, Lin Q, Li XZ. The role of PD-1 signaling in health and immune-related diseases. Front Immunol. 2023 May 16; 14:1163633.
14. Memarnejadian A, Meilleur CE, Shaler CR, Khazaie K, Bennink JR, Schell TD, Haeryfar SMM. PD-1 Blockade Promotes Epitope Spreading in Anticancer CD8+ T Cell Responses by Preventing Fratricidal Death of Subdominant Clones to Relieve Immunodomination. J Immunol. 2017 Nov 1;199(9):3348-3359.
15. Guo L, Zhang H, Chen B. Nivolumab as Programmed Death-1 (PD-1) Inhibitor for Targeted Immunotherapy in Tumor. J Cancer. 2017 Feb 10;8(3):410-416.
16. Gong J, Chehrazi-Raffle A, Reddi S, Salgia R. Development of PD-1 and PD-L1 inhibitors as a form of cancer immunotherapy: a comprehensive review of registration trials and future considerations. J Immunother Cancer. 2018 Jan 23;6(1):8.
17. Fessas P, Lee H, Ikemizu S, Janowitz T. A molecular and preclinical comparison of the PD-1-targeted T-cell checkpoint inhibitors nivolumab and pembrolizumab. Semin Oncol. 2017 Apr;44(2):136-140.
18. Bu MT, Yuan L, Klee AN, Freeman GJ. A Comparison of Murine PD-1 and PD-L1 Monoclonal Antibodies. Monoclon Antib Immunodiagn Immunother. 2022 Aug;41(4):202-209.
19. Ghareghani M, Rivest S. The Synergistic Potential of Combining PD-1/PD-L1 Immune Checkpoint Inhibitors with NOD2 Agonists in Alzheimer's Disease Treatment. Int J Mol Sci. 2023 Jun 30;24(13):10905.
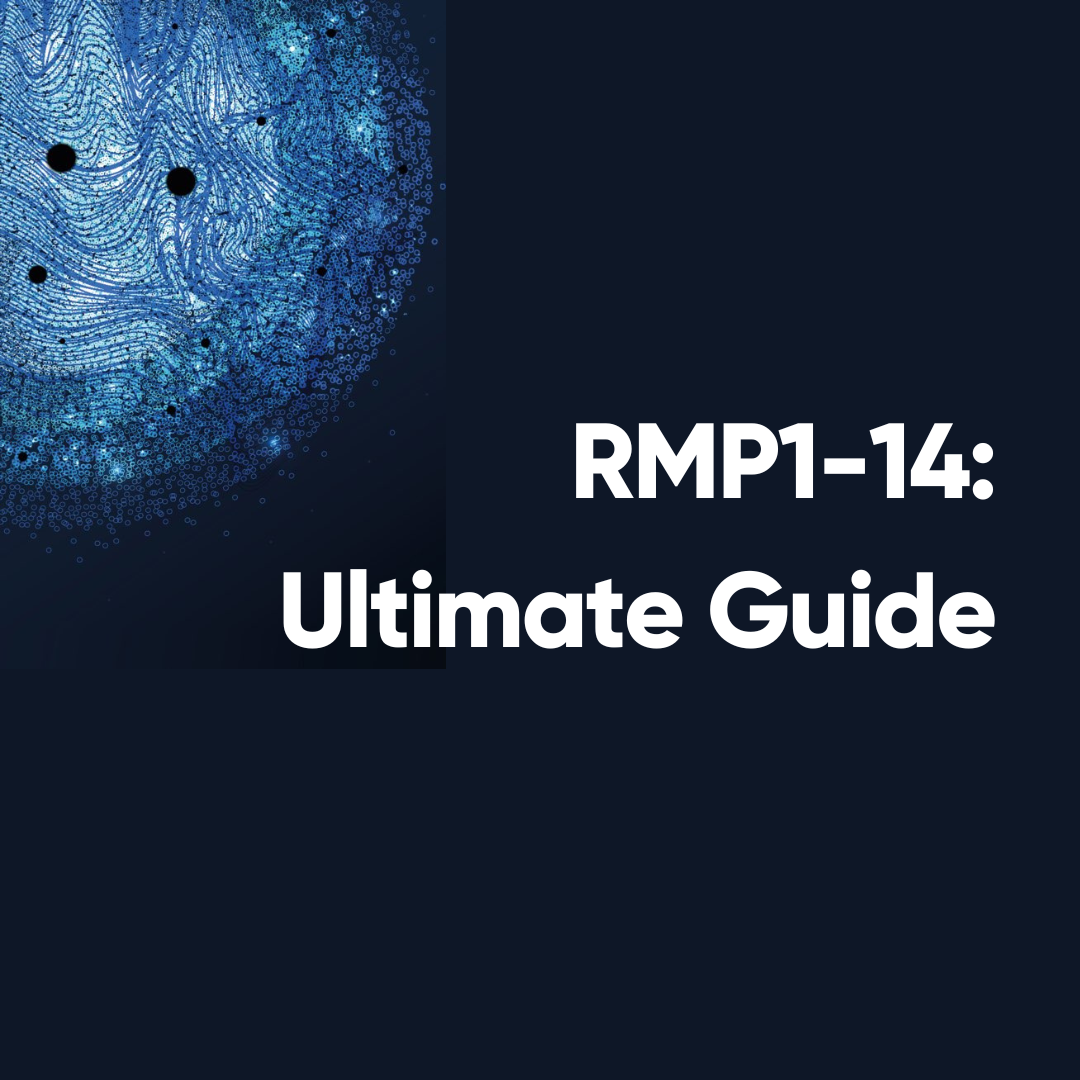
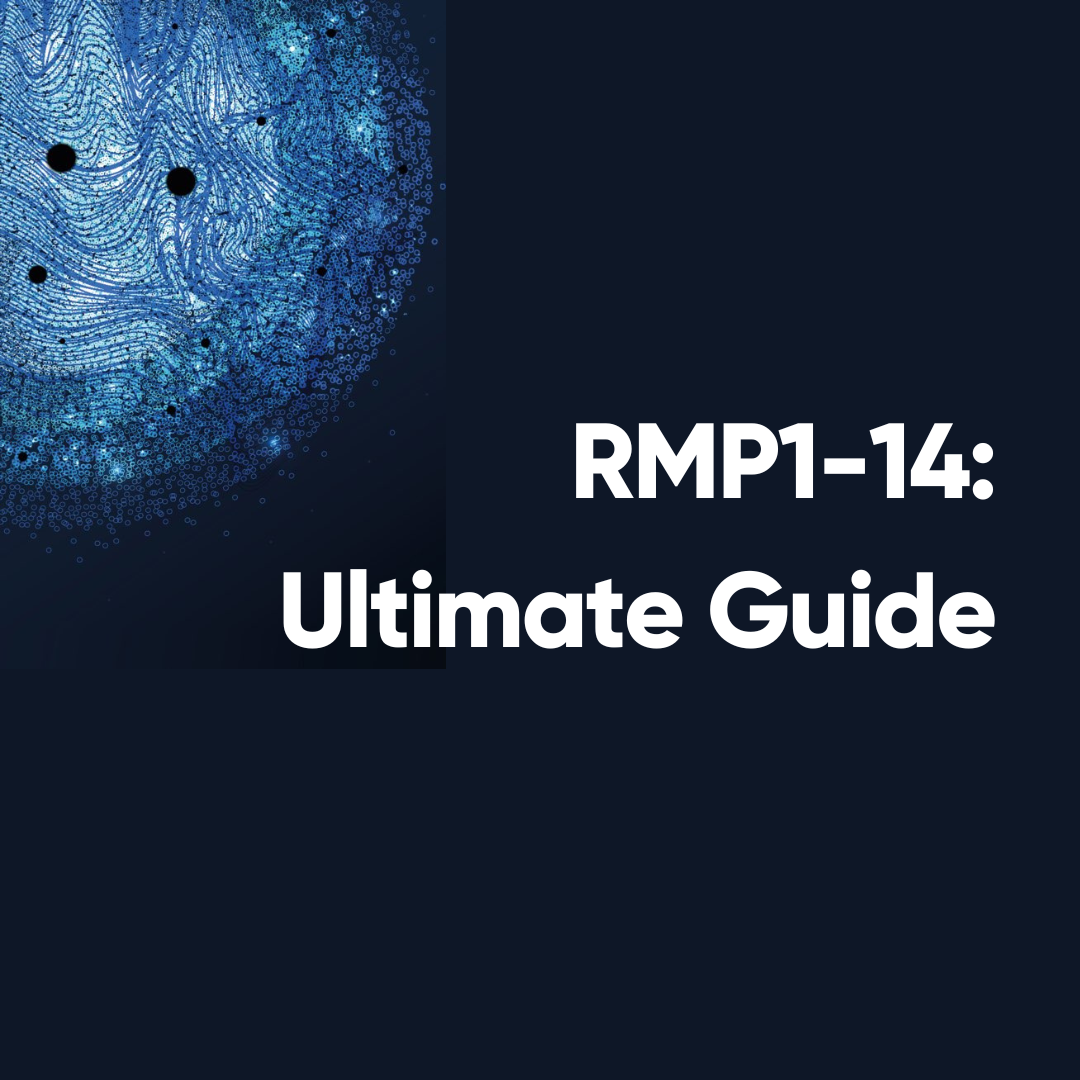
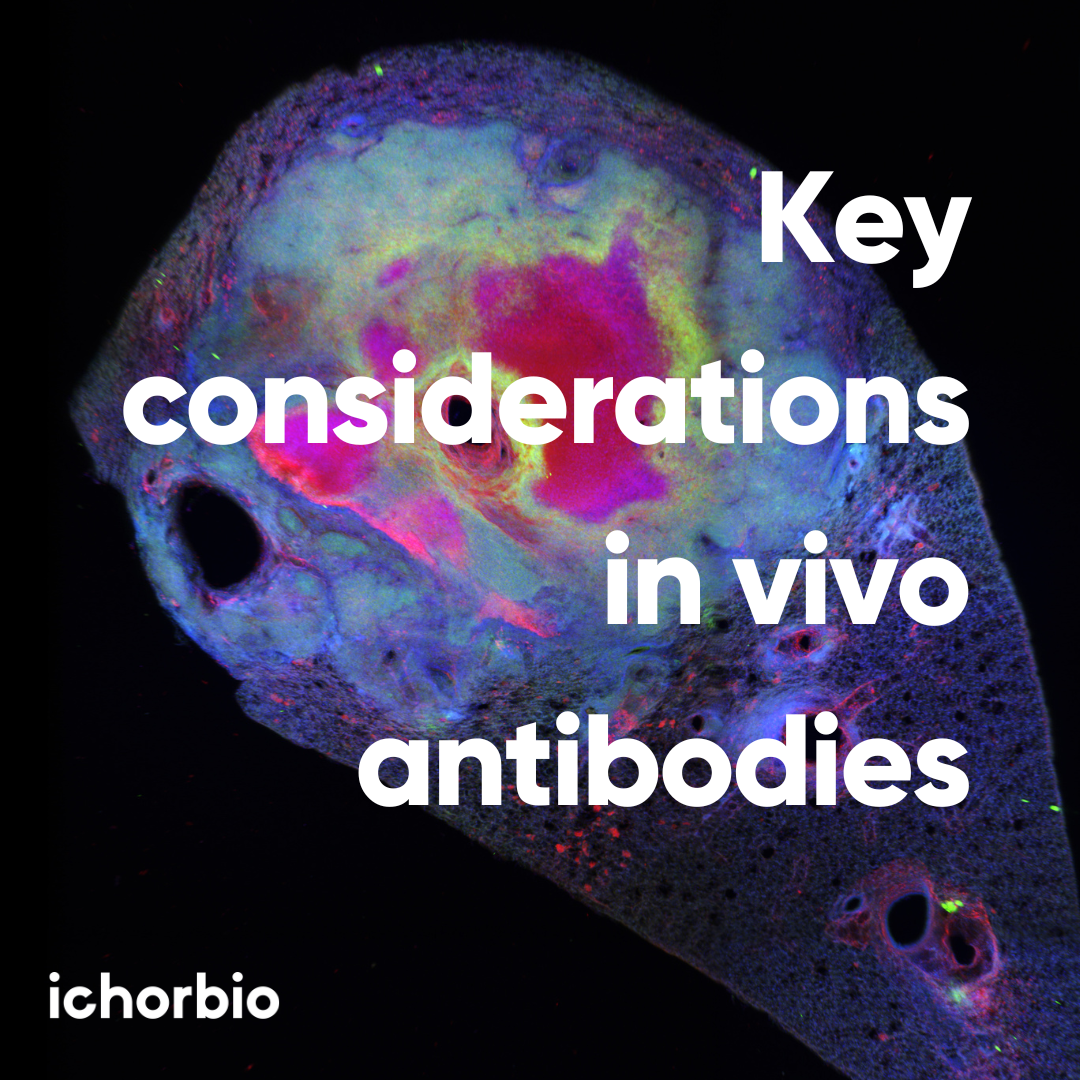
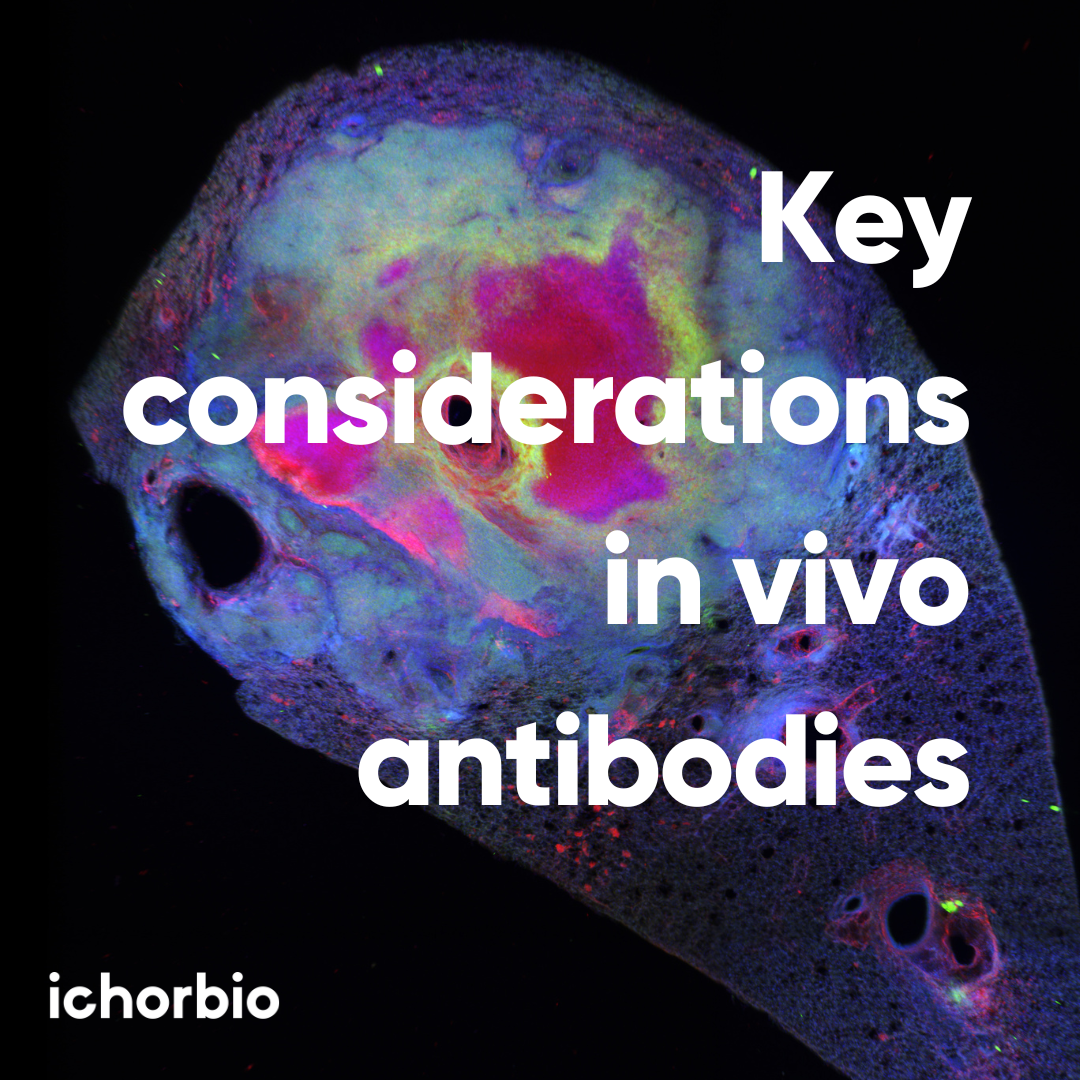
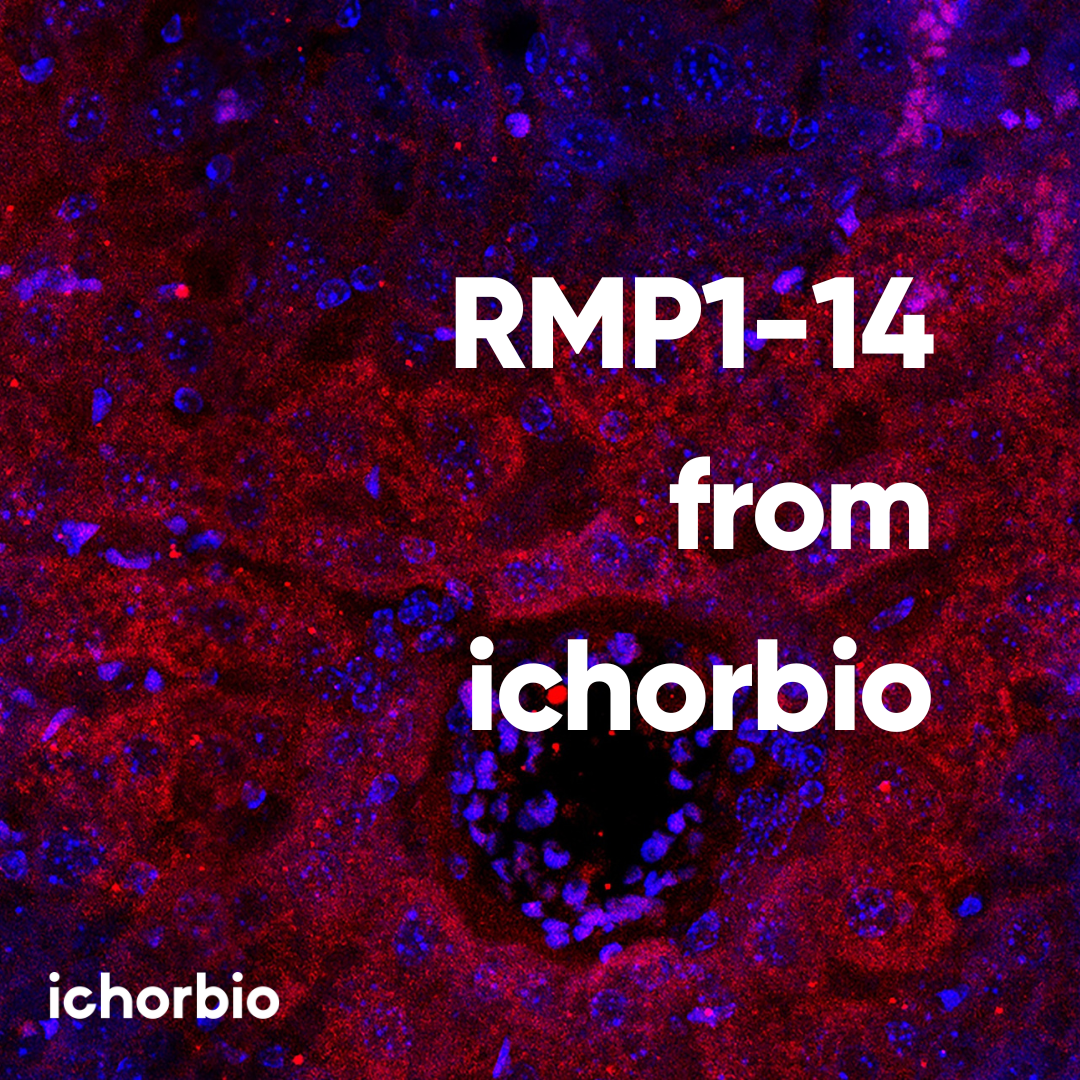
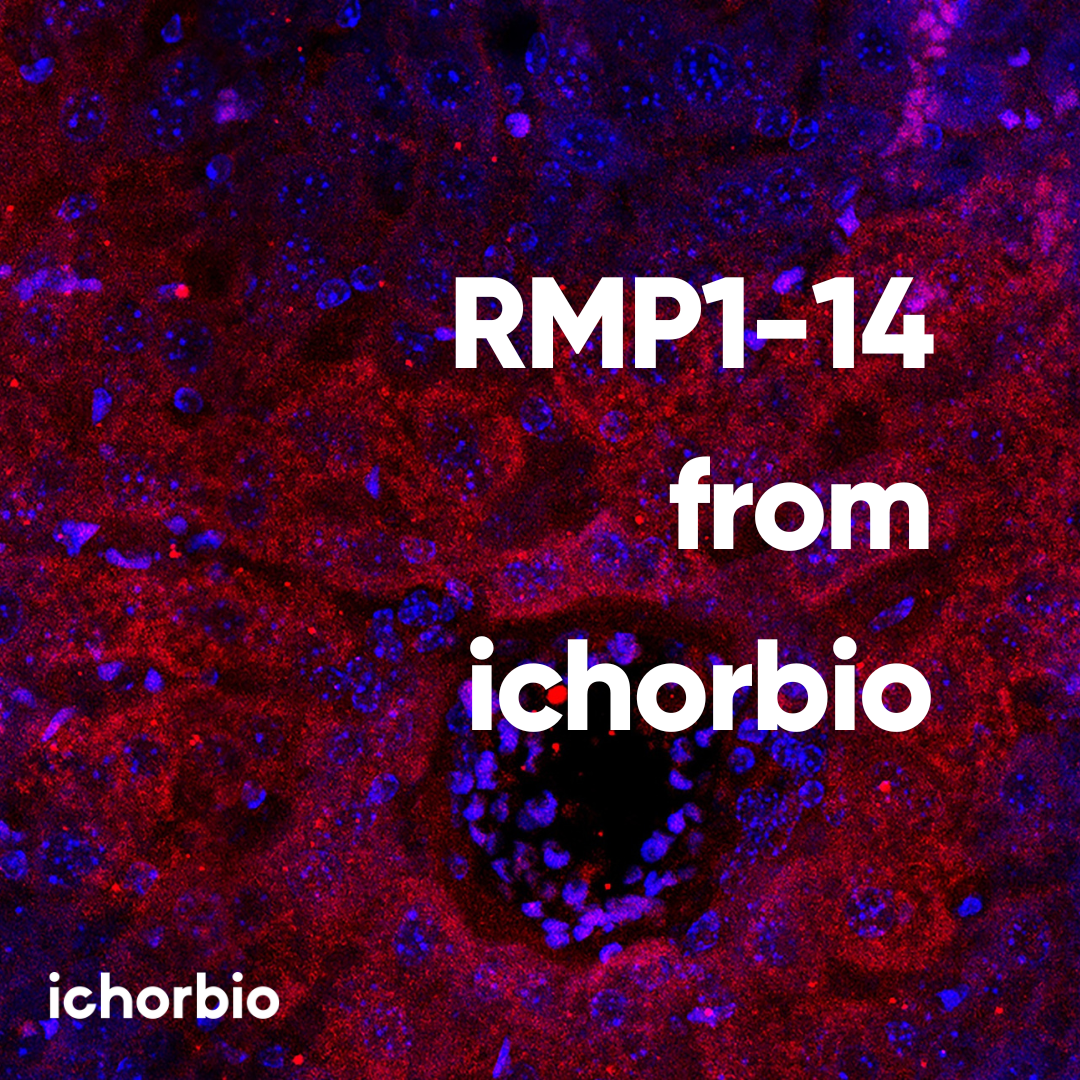
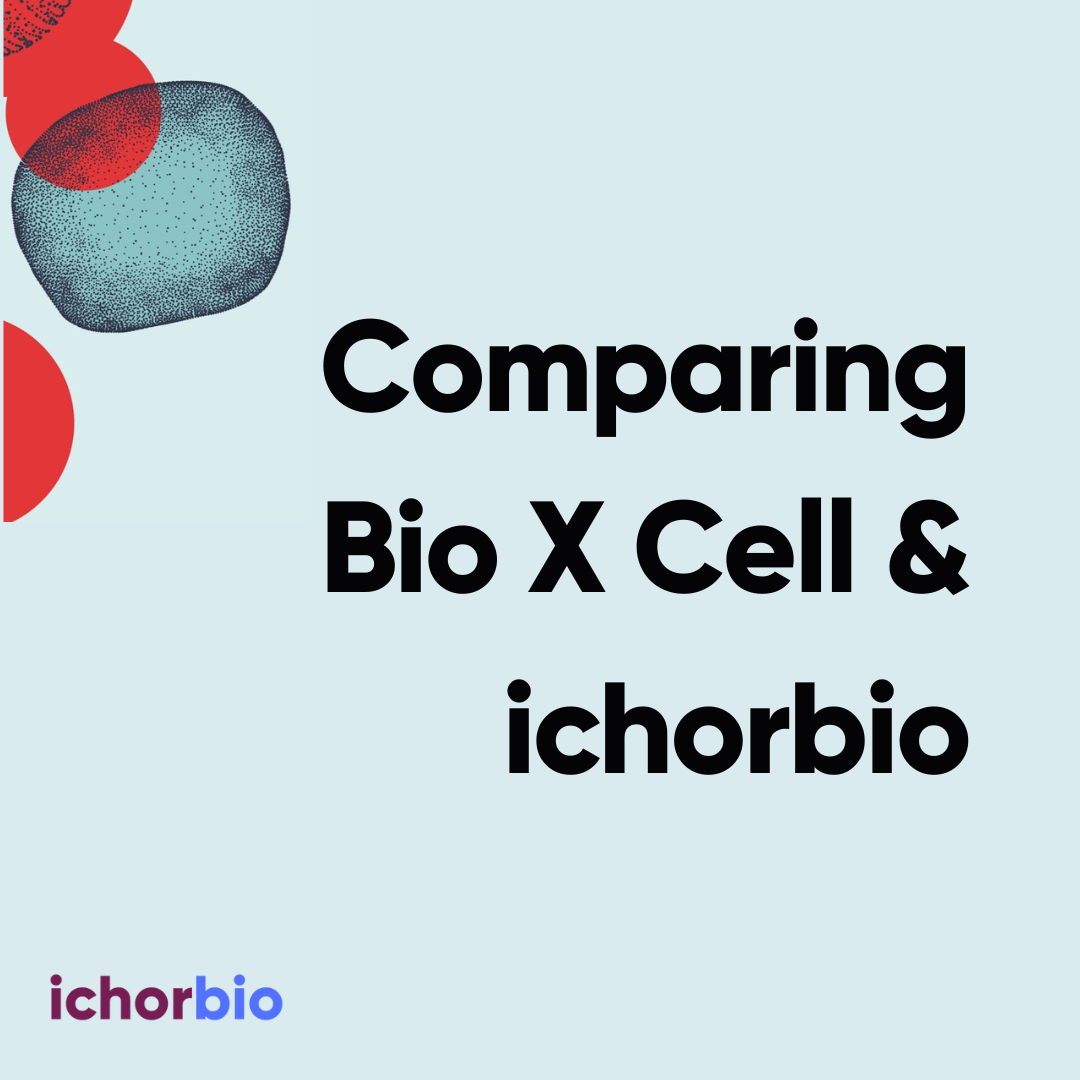
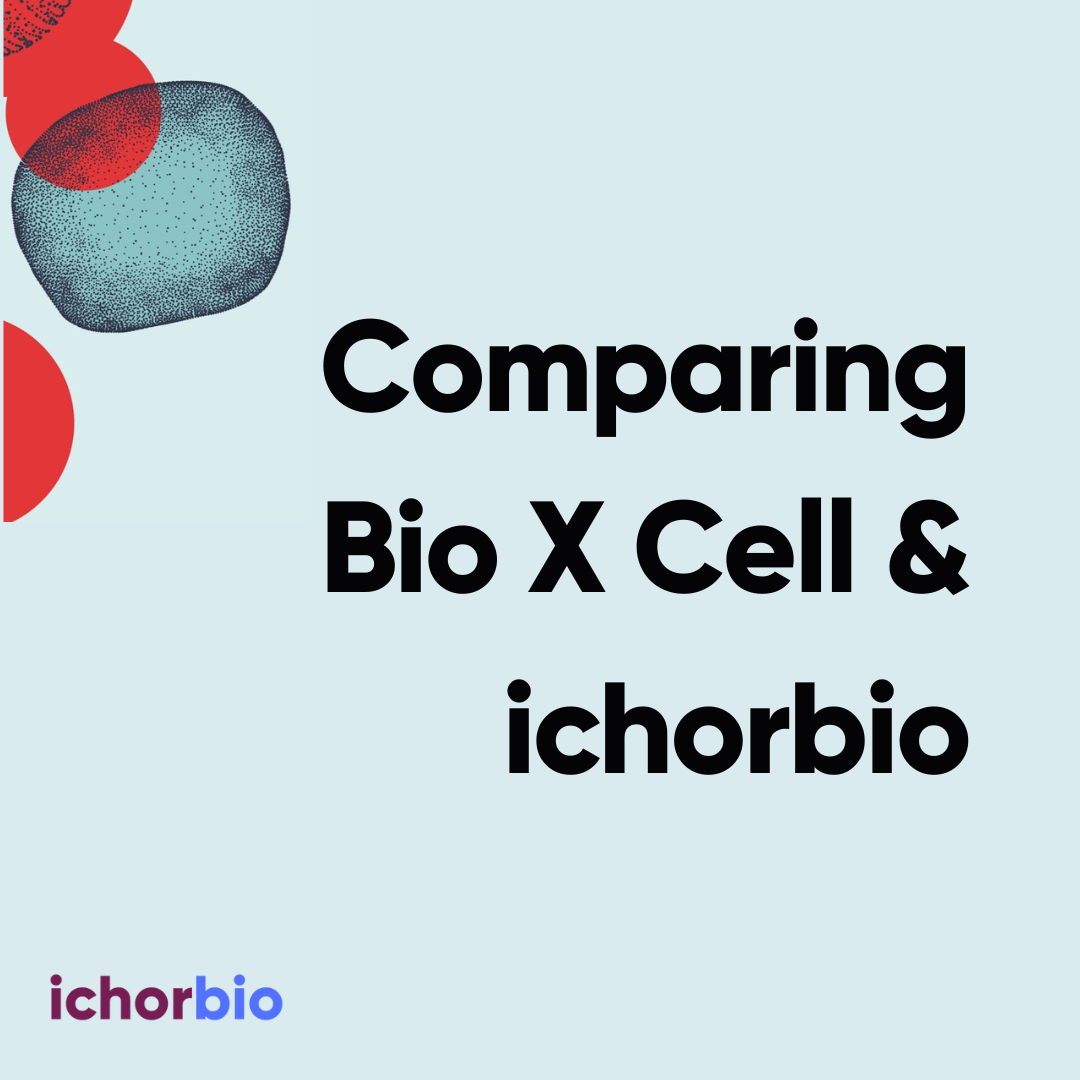