The Ultimate Guide to Using RMP1-14 for In Vivo PD-1 Blockade Studies
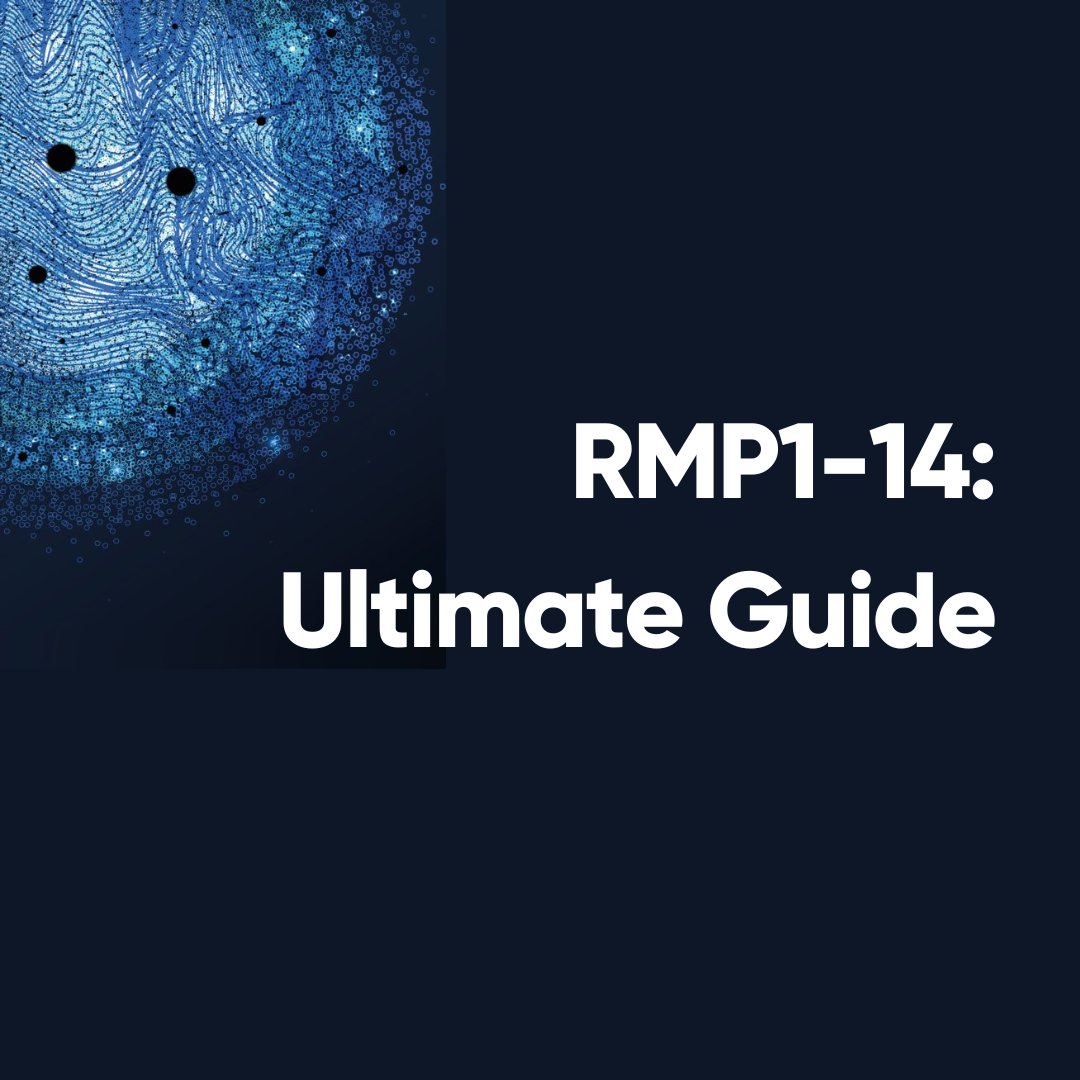
Introduction
Programmed cell death protein 1 (PD-1), an immune checkpoint protein is being used extensively as a targeted agent for developing immunotherapy for cancer patients. Its contribution to cancer and autoimmune disease development and treatment are well studied. Humanized monoclonal antibodies against PD-1 protein have proven beneficial for treating head and neck cancer patients, metastatic melanoma patients with non-small lung cancer, and renal cell carcinoma. Until now, the USA FDA has approved three ICI (immune checkpoint inhibitors), including PD-1 inhibitors, PDL-1 inhibitors (programmed cell death ligand-1), and CTLA-4 (cytotoxic T lymphocyte-associated protein 4) inhibitors) [1, 2, 3]. So, several well-established anti-PD-1 drugs, such as Cemiplimab, Nivolumab, Pembrolizumab, and RMP1-14, have developed consistency and popularity among researchers for studies of in vivo PD-1 blockade [4]. In the review, we will explore the significant landscapes of RMP1-14, in vivo recommended practices, and policies for maximizing the research results.
Action of RMP1-14
The monoclonal antibody RMP1-14, an anti-PD-1 in mice, has been designed using the hybridoma technique [5]. PD-1 is a cell surface receptor, a CD28 family member, encoded by the pdcd-1 gene in the activated T and B cells. Studies demonstrated that these immune cells treat autoimmune diseases and peripheral tolerance in mice. However, the PD-1-ligands association blocks T cell activation and cytokine releases, eventually decreasing the immune response in the living organism. The anti-PD-1, like RMP1-14, specifically interacts with the extracellular domain (immune-receptor tyrosine-based motif) of mouse PD-1, leading to RMP1-14 free from PD-L1 or PD-L2 bound [6, 7, 8, 9].
How to design RMP1-14 for in vivo studies
Several factors should be considered when designing in vivo studies with RMP1-14 to ensure optimal results. Factors such as the tumor type, immune system competency, and genetic background can all influence the response to PD-1 blockade. Primarily, selecting the appropriate animal model is crucial. Syngeneic tumor models, including MC38 colon carcinoma or B16 melanoma, are commonly used to evaluate RMP1-14 efficacy. Then, determining the optimal dosing and scheduling of RMP1-14 administration is important: typical dosing regimens range from 200-500 μg per injection, administered intraperitoneally every 3-4 days. However, the specific dosing and frequency may need to be adjusted based on the tumor model and study objectives.
When injecting antibodies into targeted mice, choosing the diluent is significant for ensuring the antibody’s stability and animals’ safety. Here are common diluents used:
- Phosphate-buffered saline (PBS): PBS is a widely used buffer because of its isotonic and non-toxic characteristics to cells. It maintains pH and osmolarity, which is essential for in vivo applications.
- Normal saline: It is another choice for diluent preparation, particularly for in vivo applications where buffering is not as critical. Saline is isotonic and keeps the physiological balance of the cells.
- Other buffer solutions: Depending on the specific application and the properties of the antibody, other buffer systems, such as Tris-buffered saline (TBS), may be used. However, it's essential to check the compatibility of these buffers with both the antibodies and the animal model.
Moreover, the buffer solution must be germ-free and free of toxic chemicals (pyrogens, endotoxin, and preservatives) that could harm the in vivo mice. It must be able to sustain the reliability and activeness of antibodies against any harm or stress for the desired animal.
Studies reported that the RMP1-14 antibody could be combined with numerous medications, such as chemotherapy, radiotherapy, protein therapy, and other immunotherapies, resulting in significant anti-tumor responses. So, during the development of these drugs, there must have been concerns about their synergistic or antagonistic effects, time optimization, and treatment schedules. Furthermore, understanding of the RMP1-14 outcomes has meaningfully been subjected to the regulations and design of the trial method. The proper control groups (isotype control antibodies or vehicle medication) must be set in the study for the accountability of non-specific things. Besides, random or blinding groups should have been used to mitigate partiality and confirm the accuracy of the data.
Recommended practices for in vivo use of RMP1-14
Some recommendations must be followed to achieve RMP1-14 success during in vivo studies. Standard storage policy and antibody preparation should be maintained. The antibody should be stored at 4°C and free from light, which stops it from degrading.
The injection process and aseptic technique must be standard during the RMP1-14 administration to prevent infection dangers and inaccurate doses. Several injection methods, such as intraperitoneal, intravenous, and intratumoral, are available, where the first one is the most common. Additionally, the efficiency of RMP1-14 depends on proper assessment and monitoring systems. Some typical parameters, including survival analysis, tumor growth dimensions, immunohistochemistry, and flow cytometry, are usually used to evaluate the PD-1 blockade performance.
Common issues for troubleshooting
Despite RMP1-14’s proven efficacy, researchers may encounter various challenges during in vivo studies. Treatment response variation can occur due to differences in tumor models, animal strains, or environmental factors. Using well-characterized models and maintaining consistent experimental conditions is essential to mitigate the variated outcomes.
Adverse effects, including autoimmunity or toxicity, can sometimes be observed with PD-1 blockade. Close animal health monitoring and appropriate management strategies may be necessary for dose reduction or treatment discontinuation.
In some cases, developing anti-drug antibodies (ADAs) against RMP1-14 can limit its efficacy over time. Strategies such as intermittent dosing or immunomodulation may help reduce the risk of ADA formation. If suboptimal responses are observed, optimization strategies may be considered for adjusting the dosing regimen, combining with other therapies, or exploring alternative administration routes.
Conclusion
The RMP1-14 is an influential antibody against PD-1’s approaches for studying in animal models and immunotherapeutic drug manufacture. Throughout the in vivo investigations, the possibility of immune-oncology advancement and valuable reagents might be stimulated, which are the most important facilities of RMP1-14.
As the field continues to evolve, new applications and opportunities for RMP1-14 are emerging, such as its use in combination with novel immunotherapies or in the study of diseases other than cancer. By staying up-to-date with the latest developments and sharing insights within the scientific community, we can collectively harness the power of RMP1-14 to drive meaningful progress in immunotherapy research. The key advantages of RMP1-14 for in vivo use are its proven efficacy across a wide range of tumor models and its well-established dosing and administration protocols. Furthermore, RMP1-14 is commercially available from multiple suppliers, ensuring researchers' ease of access and reproducibility.
Are you seeking reasonable, high-quality RMP1-14 antibodies to further your research? Ichorbio has an extensive reputation for providing various stages of high-grade RMP1-14 antibodies, such as low endotoxin, ultra-low endotoxin, extremely low endotoxin, murine versions, and Fc silenced versions.
To find the RMP1-14 products: https://ichor.bio/rmp1-14
References:
1. Shiravand Y, Khodadadi F, Kashani SMA, Hosseini-Fard SR, Hosseini S, Sadeghirad H, Ladwa R, O'Byrne K, Kulasinghe A. Immune Checkpoint Inhibitors in Cancer Therapy. Curr Oncol. 2022 Apr 24;29(5):3044-3060.
2. Zam W, Ali L. Immune Checkpoint Inhibitors in the Treatment of Cancer. Curr Rev Clin Exp Pharmacol. 2022;17(2):103-113.
3. Bernardo M, Tolstykh T, Zhang YA, Bangari DS, Cao H, Heyl KA, Lee JS, Malkova NV, Malley K, Marquez E, Pollard J, Qu H, Roberts E, Ryan S, Singh K, Sun F, Wang E, Bahjat K, Wiederschain D, Wagenaar TR. An experimental model of anti-PD-1 resistance exhibits activation of TGFß and Notch pathways and is sensitive to local mRNA immunotherapy. Oncoimmunology. 2021 Mar 16;10(1):1881268.
4. Agrawal K, Hill RC, Wilkinson BL, Allison PB, Thomas CE. Quantification of the anti-murine PD-1 monoclonal antibody RMP1-14 in BALB/c mouse plasma by liquid chromatography-tandem mass spectrometry and application to a pharmacokinetic study. Anal Bioanal Chem. 2020 Jan;412(3):739-752.
5. Zhai Y, Dong S, Li H, Zhang Y, Shami P, Chen M. Antibody-mediated depletion of programmed death 1-positive (PD-1+) cells. J Control Release. 2022 Sep; 349:425-433.
6. Bu MT, Yuan L, Klee AN, Freeman GJ. A Comparison of Murine PD-1 and PD-L1 Monoclonal Antibodies. Monoclon Antib Immunodiagn Immunother. 2022 Aug;41(4):202-209. doi: 10.1089/mab.2021.0068. Epub 2022 Aug 4.
7. Liu H, Liu Y, Zhao Z, Li Y, Mustafa B, Chen Z, Barve A, Jain A, Yao X, Li G, Cheng K. Discovery of Anti-PD-L1 Human Domain Antibodies for Cancer Immunotherapy. Front Immunol. 2022 Apr 4; 13:838966. doi: 10.3389/fimmu.2022.838966.
8. https://www.thermofisher.com/antibody/product/CD279-PD-1-Antibody-clone-RMP1-14-Monoclonal/14-9982-82 [Extracted information on May 21, 2024]
9. Yamazaki T, Akiba H, Koyanagi A, Azuma M, Yagita H, Okumura K. Blockade of B7-H1 on macrophages suppresses CD4+ T cell proliferation by augmenting IFN-gamma-induced nitric oxide production. J Immunol. 2005 Aug 1;175(3):1586-92.
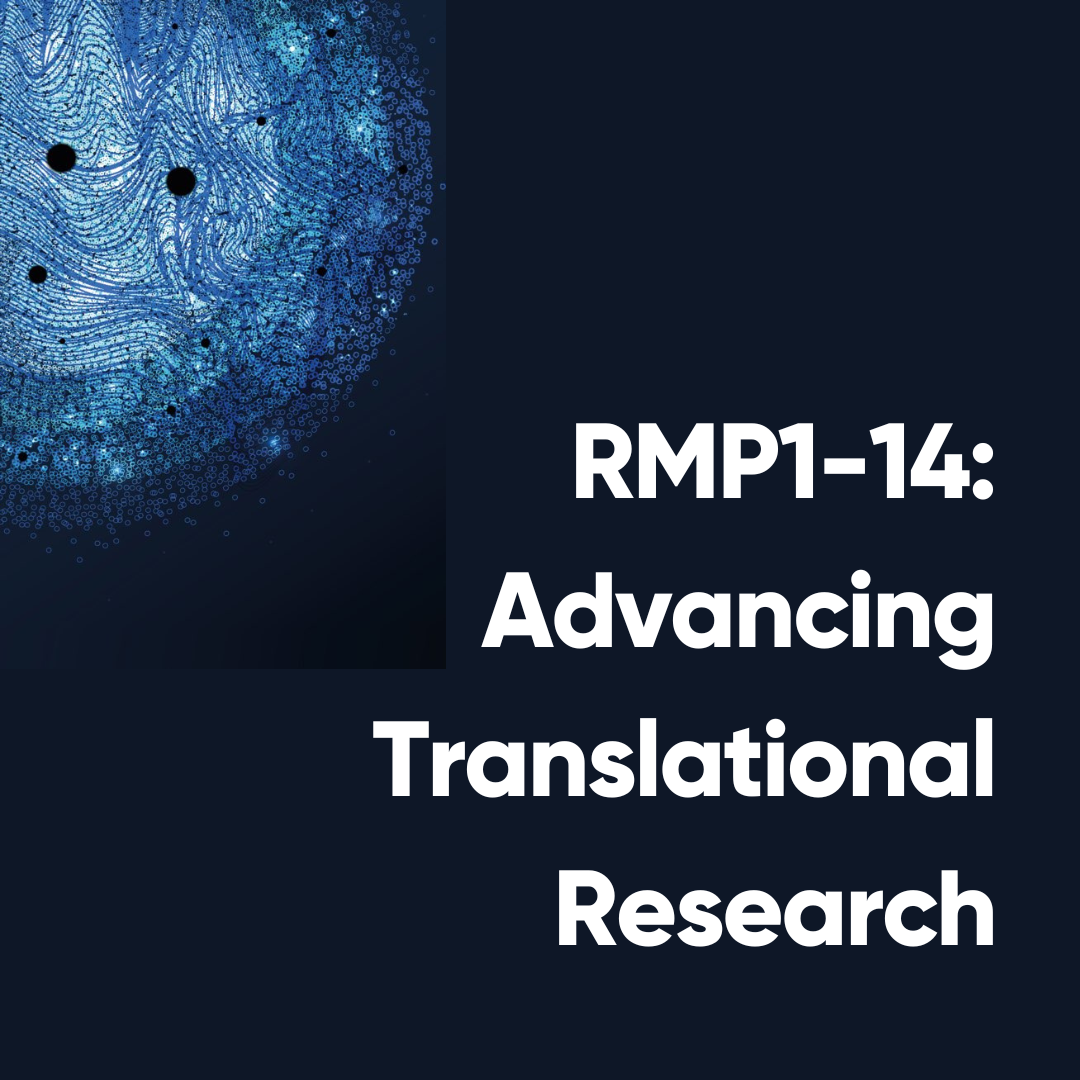
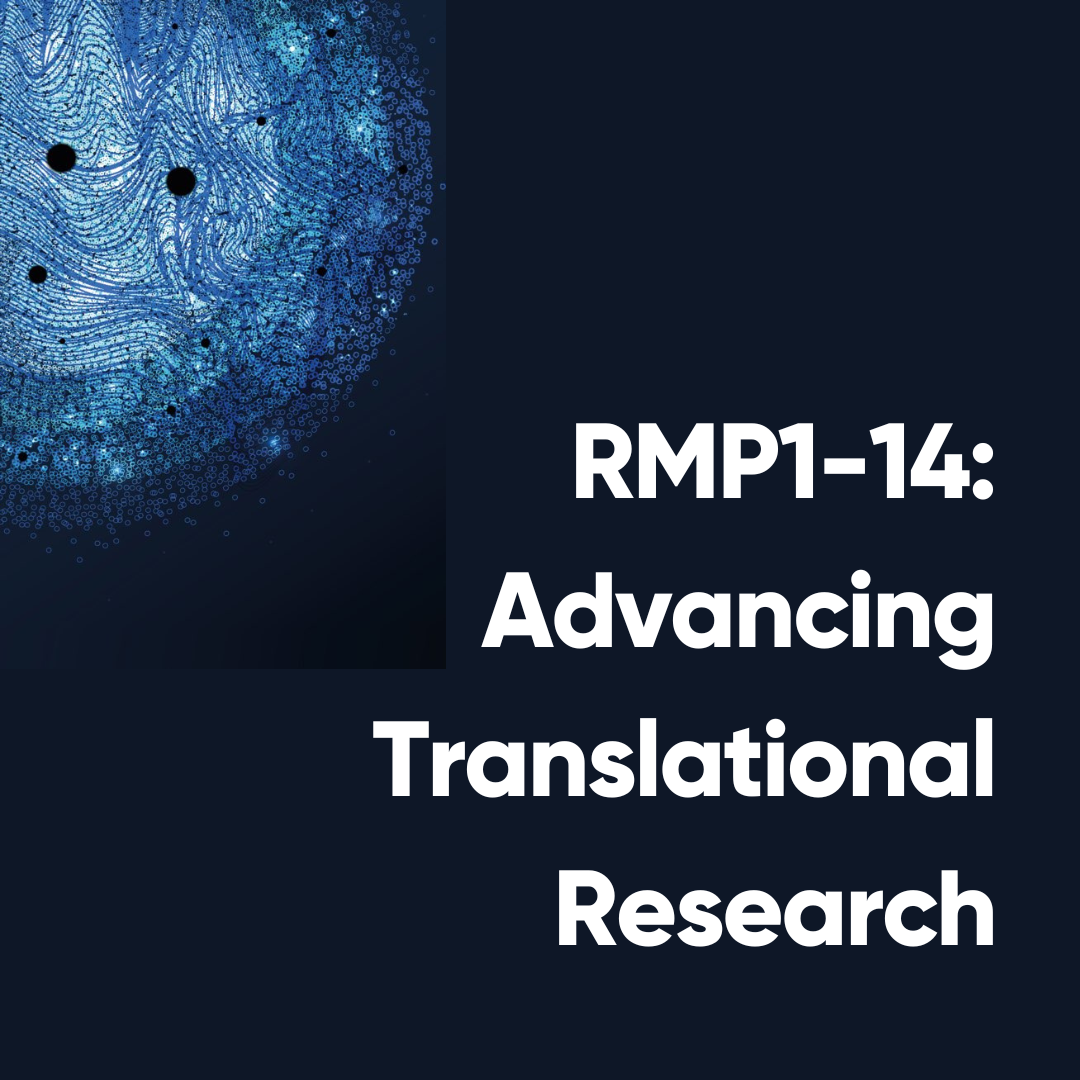
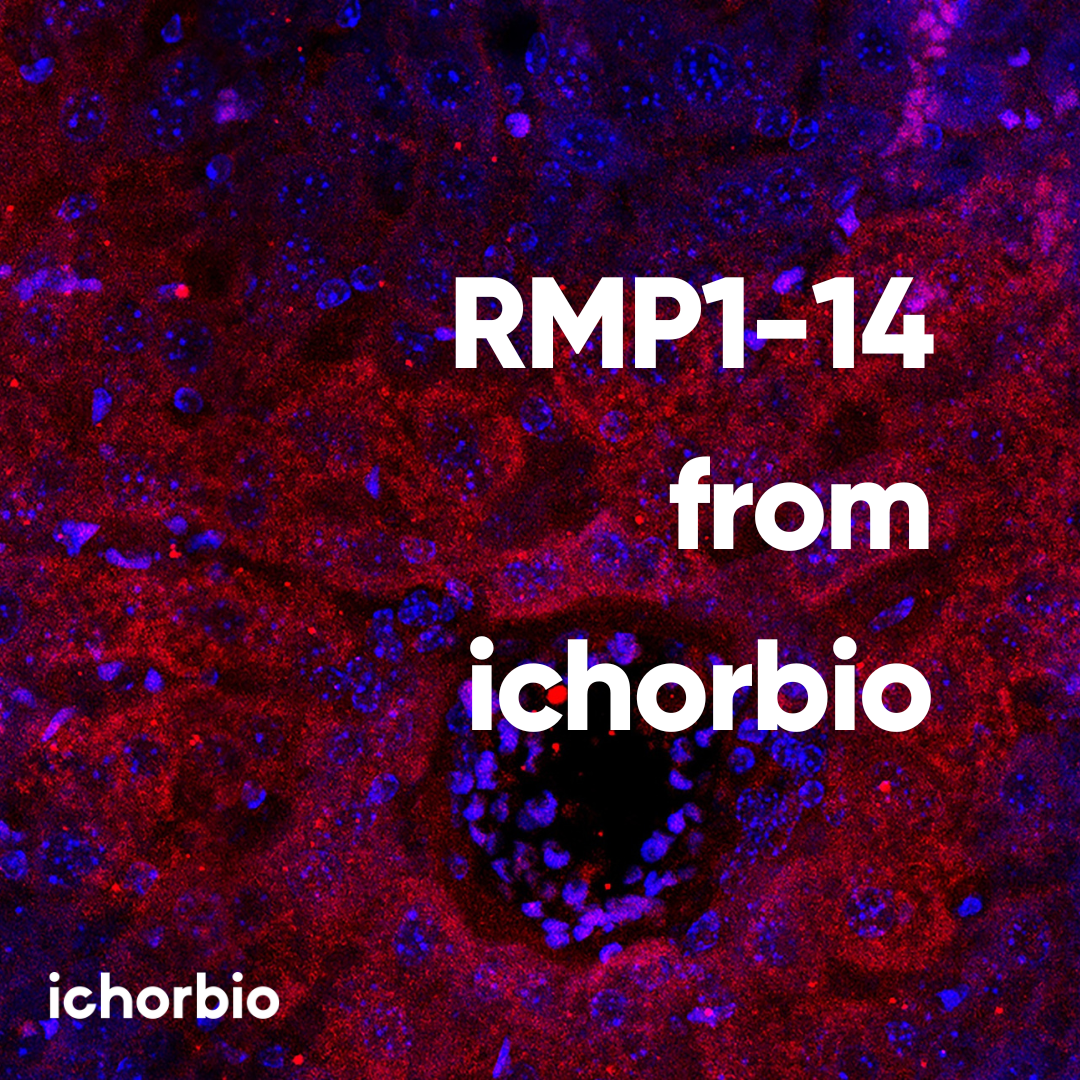
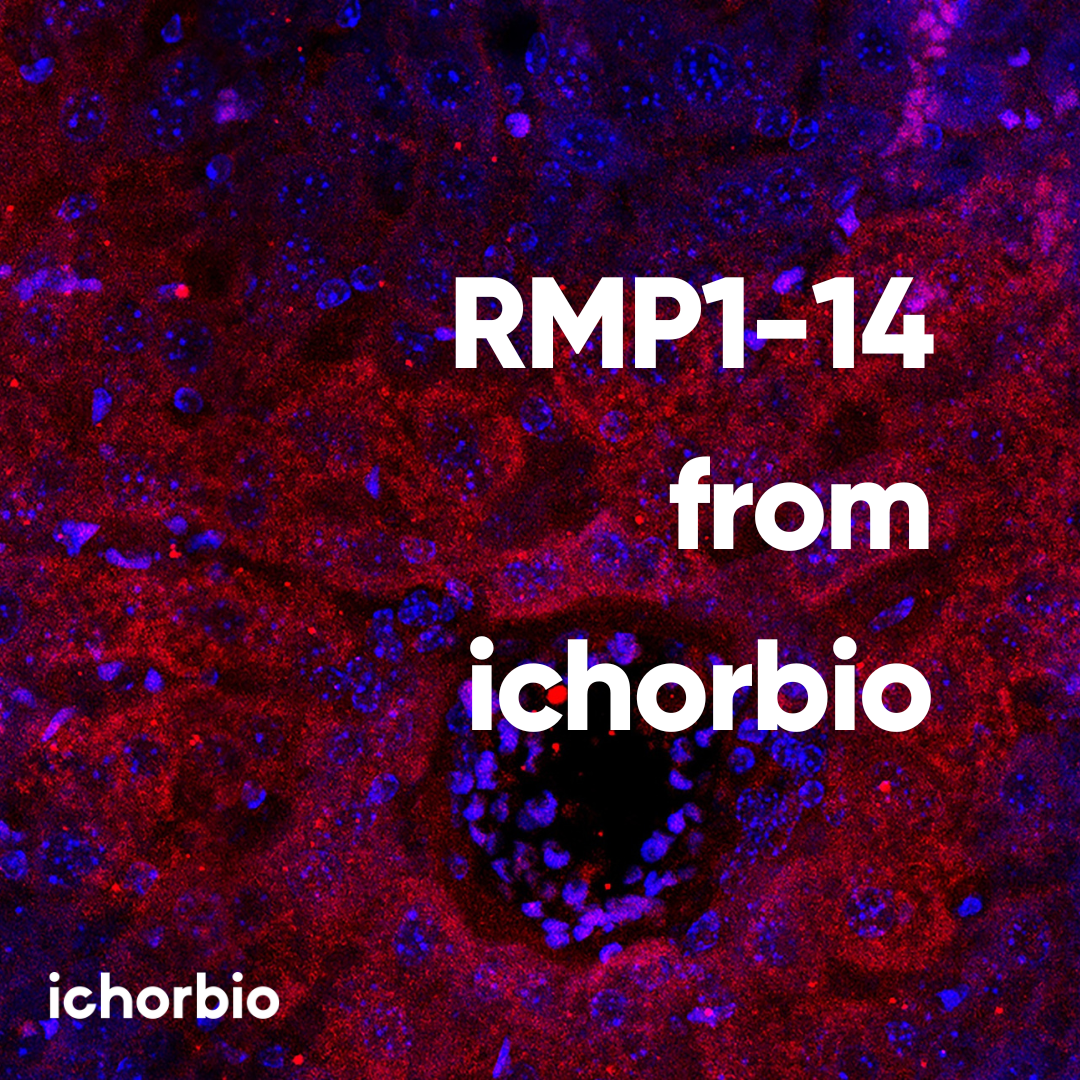
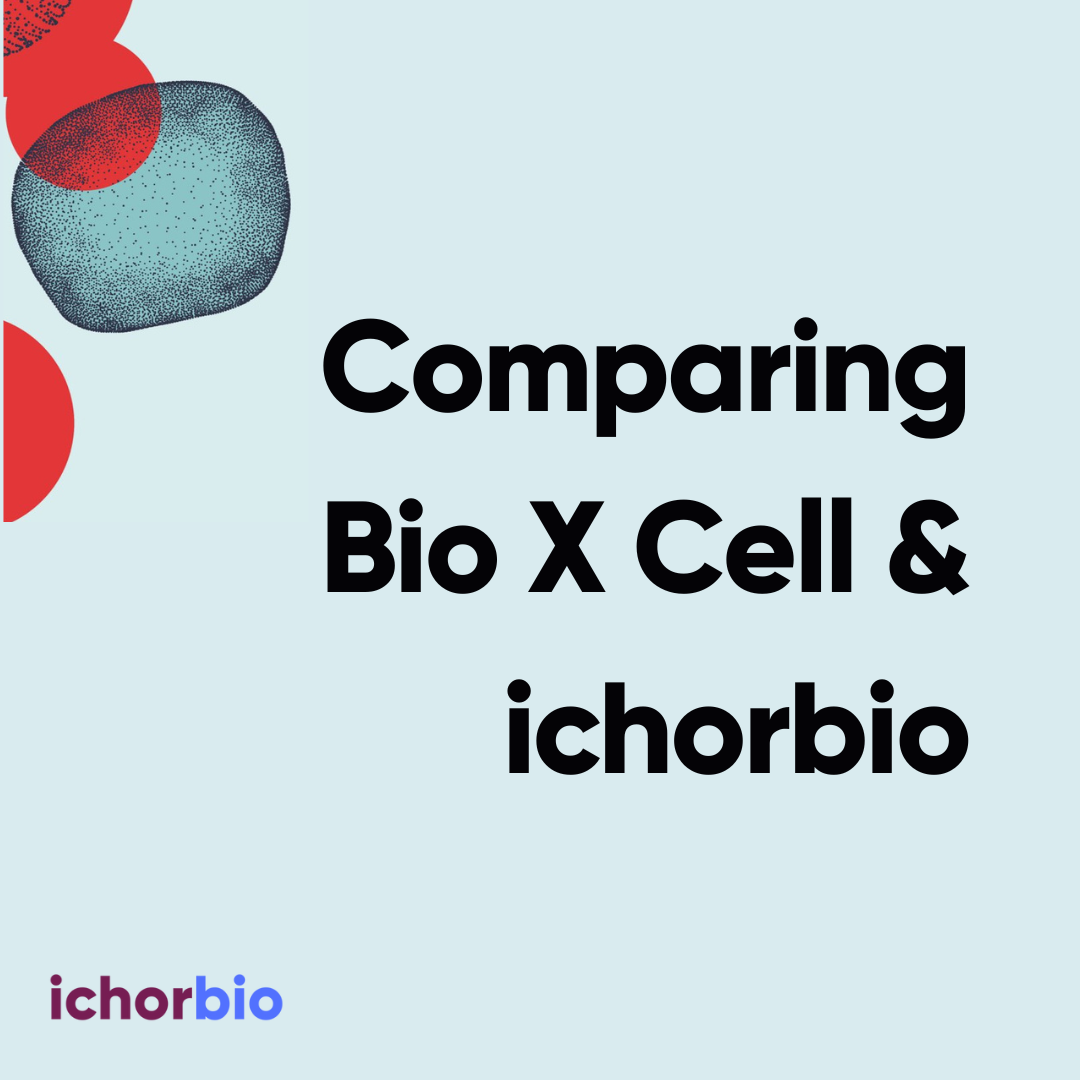
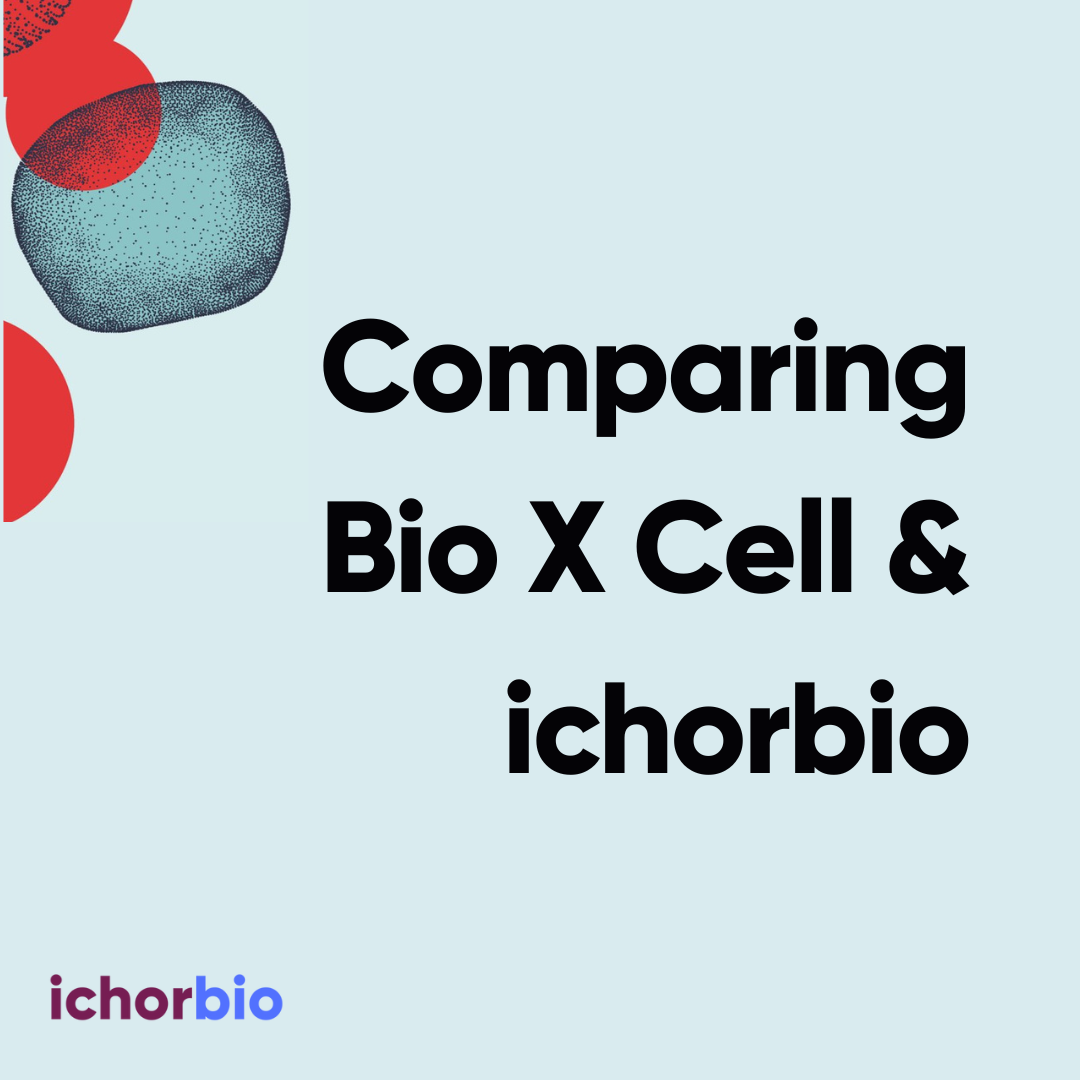
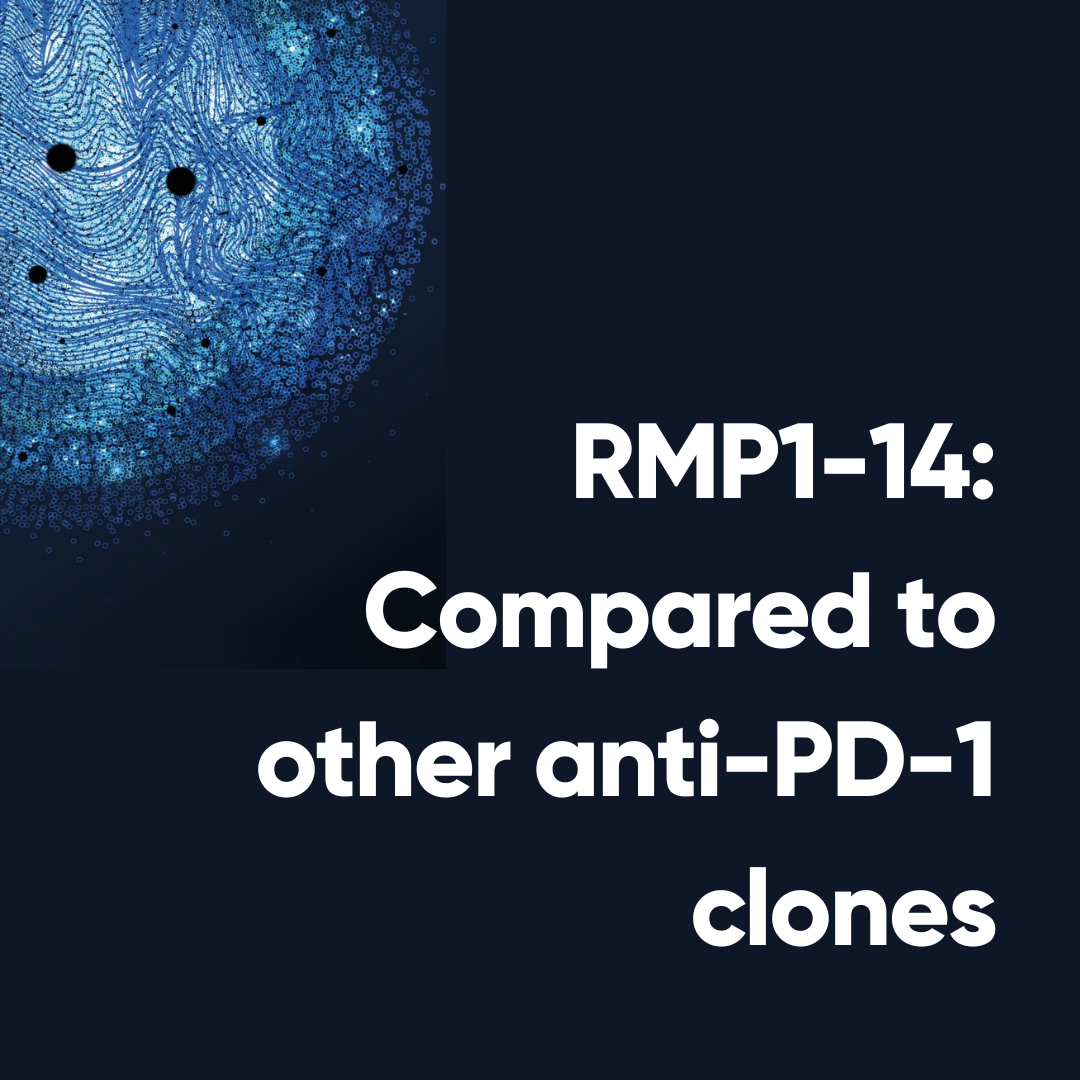
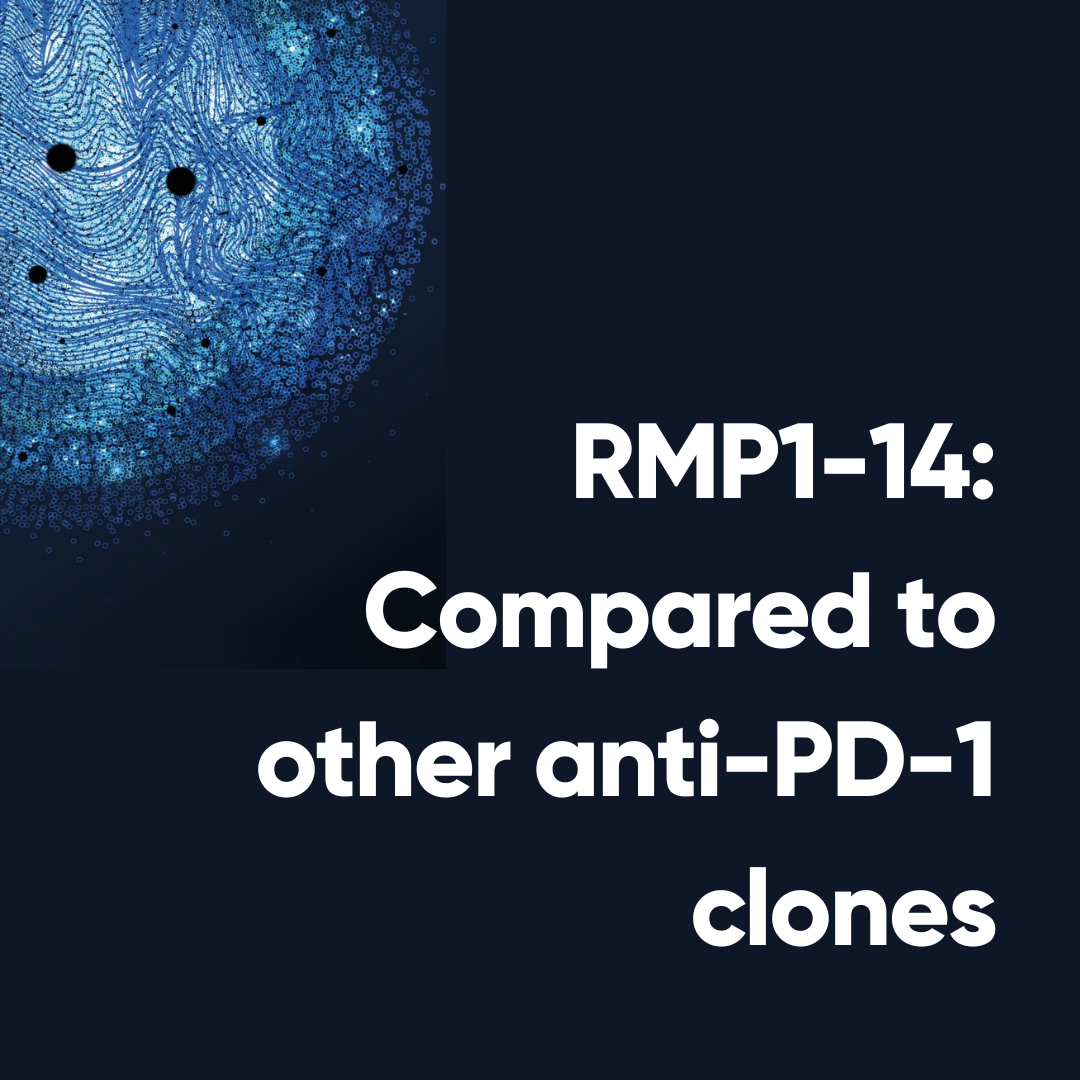